Can water be simultaneously solid and liquid?
Yes, according to new research from University of Rochester scientists. The Rochester researchers, along with their colleagues at the Lawrence Livermore National Laboratory in California, used lasers at Rochester’s Laboratory for Laser Energetics (LLE) to flash-freeze water into an exotic water ice phase. Using X-ray diffraction, they were able to identify and record, for the first time, the ice’s atomic structure. Their research, published in the journal Nature, may give more insight into the interior structures of giant planets in our galaxy.
“Icy giants like Uranus and Neptune consist of about two-thirds water by mass,” says Ryan Rygg, an assistant professor of mechanical engineering and of physics and a senior scientist at the LLE, who worked on the project. “I expect the experimental confirmation of this exotic phase of water will push planetary scientists to overhaul their picture of the interiors of these planets.”
In 1998, scientists first predicted that water would transition to an exotic state when it was subjected to extreme pressures and temperatures—similar to the conditions that exist in planets such as Uranus and Neptune. In 2018, the Rochester and Livermore team presented the first experimental evidence for this exotic state of water, known as superionic ice, that is simultaneously a solid and a liquid because it is composed of oxygen atoms in a solid crystalline lattice and liquid-like hydrogen. However, the team was only able to observe general properties of the ice, such as temperature and energy.
Now, using laser-driven shockwaves and X-ray diffraction, the researchers not only created superionic ice, but recorded images of the microscopic crystalline structure of water in the superionic ice phase—all in a few billionths of a second.
“We wanted to determine the atomic structure of superionic water,” says Federica Coppari, a physicist at Livermore. “But given the extreme conditions at which this elusive state of matter is predicted to be stable, compressing water to such pressures and temperatures and simultaneously taking snapshots of the atomic structure was an extremely difficult task, which required an innovative experimental design.”
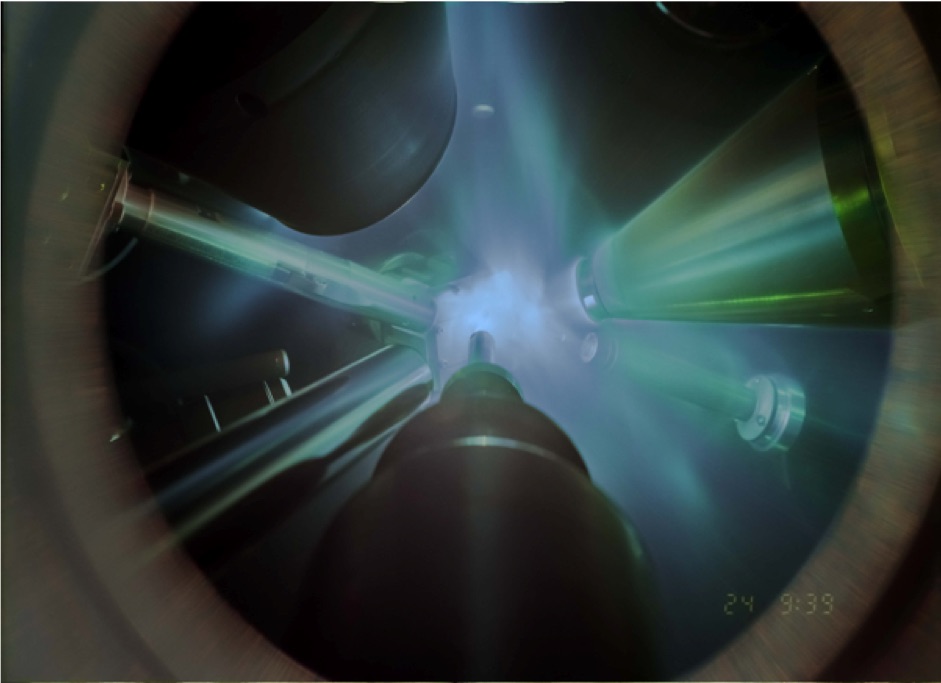
In order to flash freeze water into the superionic ice phase, the researchers used six giant laser beams of progressively increasing intensity to compress a thin layer of liquid water to extreme pressures—one-to-four million times the pressure of Earth’s atmosphere—and extreme temperatures of 3,000-to-5,000 degrees Fahrenheit. Even though it is under extreme temperatures, when the water is also under extreme pressures, it freezes into the superionic water ice phase. As researchers apply more pressure to water, the resulting ice can have many different crystalline structures. Scientists refer to the different types of ice as ice I, ice II, and so on up through ice XVII. They proposed to call the new superionic ice “ice XVIII.”
By generating X-rays precisely timed to illuminate the compressed water sample, the researchers were able to observe the dense ice crystals that formed at the atomic level.
Although superionic ice doesn’t exist naturally on Earth, recreating it in the lab and better understanding its atomic structure will give researchers more insight into water-rich planets like Uranus and Neptune and other icy giants in our galaxy.